Enzymes/Biological catalysts
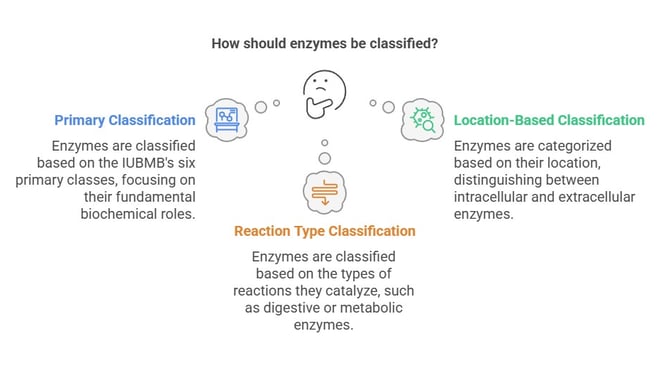
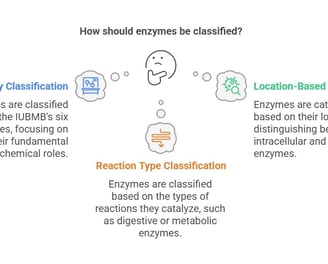
What Are Enzymes?
Enzymes are primarily proteins that facilitate biochemical reactions without being consumed during the process.
They achieve this by lowering the activation energy required for reactions, thus increasing reaction rates.
Each enzyme is specific to a particular reaction or group of related reactions, ensuring precise control over the various biochemical processes in the body.
Structure of Enzymes
Enzymes are composed of amino acid chains folded into intricate three-dimensional structures. This unique conformation creates an active site, a specialized region where substrate molecules bind and undergo chemical transformation. The specificity of an enzyme is largely determined by the shape and chemical environment of its active site.
Some enzymes require additional non-protein components to function effectively:
Cofactors: Inorganic ions (e.g., Fe²⁺, Mg²⁺) that assist in enzyme activity.
Coenzymes: Organic molecules, often derived from vitamins, that participate in the enzymatic reaction.
The protein portion of an enzyme, without its cofactor or coenzyme, is termed an apoenzyme and is inactive. When combined with its non-protein component, it forms a holoenzyme, the active form.
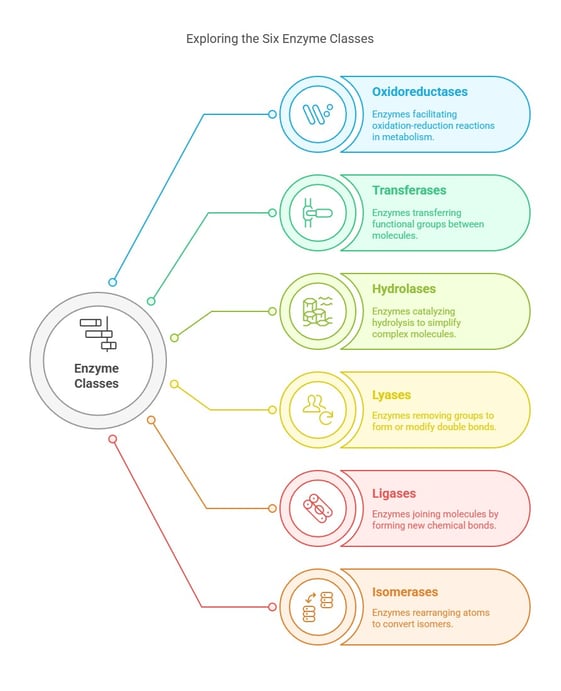
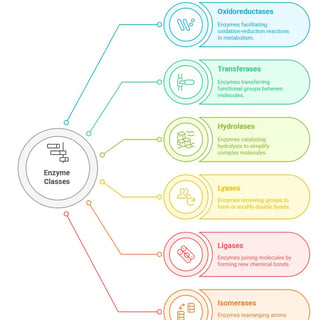
2. Transferases (EC 2)
Transferases catalyze the transfer of functional groups (such as methyl or phosphate groups) from one molecule to another. They play a significant role in various biosynthetic pathways.
Functions:
Transferases catalyze the transfer of a specific functional group (such as a methyl, glycosyl, or amino group) from a donor molecule to an acceptor molecule.
A quintessential example is the group of transaminases, such as aspartate aminotransferase (AST) and alanine aminotransferase (ALT), which are pivotal in amino acid metabolism. Increased ALT levels are commonly associated with liver dysfunction.
Significance in Medicine:
In the clinical setting, the activity of transferases—especially AST and ALT—is directly linked to liver function. Elevated levels often indicate liver injury or disease. Moreover, other transferases are intimately linked with nucleotide, carbohydrate, and lipid metabolism, underscoring their relevance in metabolic disorders and as potential targets for therapeutic intervention.
1. Oxidoreductases (EC 1)
These enzymes facilitate oxidation-reduction reactions, where electrons are transferred between molecules. They are crucial in metabolic pathways like cellular respiration.
Functions:
Oxidoreductases catalyze oxidation-reduction (redox) reactions. They facilitate the transfer of electrons from one molecule (the reductant) to another (the oxidant). Key types include dehydrogenases, oxidases, and reductases.
For example, lactate dehydrogenase (LDH) plays a critical role in anaerobic glycolysis by interconverting lactate and pyruvate, while cytochrome oxidases are essential in the electron transport chain for ATP production. Elevated LDH levels in the blood can indicate tissue damage or disease.
Significance in Medicine:
Defects or altered activities in oxidoreductases can result in impaired energy metabolism and oxidative stress. Enzyme assays measuring levels of these enzymes (e.g., LDH) are commonly used in clinical diagnostics to evaluate tissue damage, including myocardial infarction and liver diseases. Abnormal redox balances have also been linked with several pathologies, including neurodegenerative disorders and cancer.
Classification of Enzymes
Enzymes are indispensable biological catalysts that accelerate chemical reactions within the body, ensuring that vital physiological processes occur efficiently. Understanding the classification of enzymes is fundamental in medical biochemistry as it provides insights into their diverse functions and clinical significance.
The International Union of Biochemistry and Molecular Biology (IUBMB) classifies enzymes into six primary categories based on the type of reaction they catalyze. While the six classes comprise the primary classification system endorsed by the IUBMB, enzymes can also be categorized based on their location (e.g., intracellular vs. extracellular) or the type of reactions they catalyze in broader terms (such as digestive enzymes vs. metabolic enzymes). For instance, extracellular enzymes, like those secreted by the pancreas, are vital for digestion, whereas intracellular enzymes drive metabolic reactions within cells.
6. Ligases (EC 6)
Ligases facilitate the joining of two molecules by forming new chemical bonds, typically coupled with the hydrolysis of ATP. They are crucial in DNA replication and repair.
Functions:
Ligases are enzymes that catalyze the joining of two molecules, coupling the formation of a new chemical bond with the hydrolysis of ATP (or another nucleoside triphosphate).
DNA ligase, which joins DNA strands during replication and repair, is a well-known example. Similarly, aminoacyl-tRNA synthetases attach amino acids to their corresponding tRNA molecules, a critical step in protein synthesis.
Significance in Medicine:
Many ligases are fundamental to cellular maintenance and replication. DNA ligases, for instance, are targets in chemotherapy because inhibiting them can prevent cancer cells from repairing their DNA. Inherited defects in these enzymes may also lead to genomic instability and are studied in the context of genetic disorders and aging.
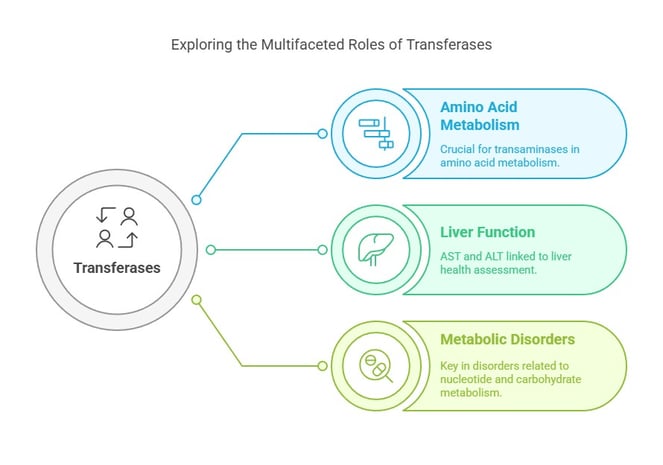
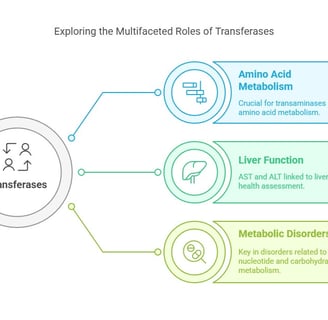
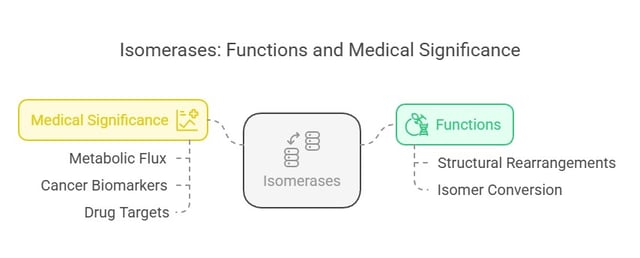
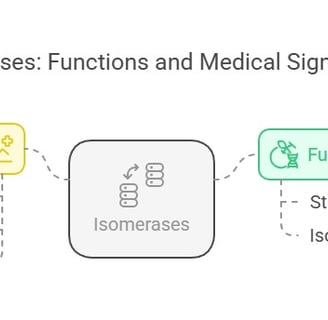
3. Hydrolases (EC 3)
These enzymes catalyze the hydrolysis of chemical bonds, breaking down complex molecules into simpler ones by adding water. They are essential in the digestive processes
Functions:
Hydrolases catalyze hydrolytic reactions, where the addition of water breaks chemical bonds. This class includes proteases, lipases, nucleases, and esterases. Such enzymes are broad in function: proteases in protein digestion, lipases in fat digestion, and nucleases in nucleic acid metabolism.
For example, Amylase breaks down starch into simple sugars like maltose and dextrin. Elevated amylase levels can indicate pancreatic disorders.
Significance in Medicine:
Beyond digestion, many hydrolases play roles in cellular recycling and signal regulation. For instance, lysosomal hydrolases break down macromolecules within cells, and deficiencies in these enzymes are linked to lysosomal storage disorders like Gaucher’s or Tay-Sachs disease. Their clinical measurement aids in diagnosing such complex metabolic disorders.
4. Lyases (EC 4)
Lyases remove groups from substrates without hydrolysis, often forming double bonds or adding groups to double bonds. They are involved in various metabolic pathways.
Functions:
Lyases facilitate the breaking of various chemical bonds by means other than hydrolysis and oxidation, often forming a new double bond or a new ring structure in the process. They typically work by removing groups from substrates, as seen in decarboxylases and dehydratases.
An example is fumarase in the citric acid cycle, which catalyzes the reversible hydration of fumarate to malate. Pyruvate decarboxylase converts pyruvate to acetaldehyde and CO₂ in alcoholic fermentation.
Significance in Medicine:
These enzymes are critical in intermediary metabolism, and any disruption in their function could lead to metabolic imbalances. Defects in lyase activity can compromise energy production or lead to the accumulation of intermediate metabolites, which can be detrimental in conditions like certain inborn errors of metabolism. Understanding lyase mechanisms is also valuable in designing inhibitors for therapeutic purposes.
5. Isomerases (EC 5)
Isomerases catalyze the rearrangement of atoms within a molecule, converting one isomer into another. This function is vital in metabolic pathways that require specific isomer forms.
Functions:
Isomerases facilitate structural rearrangements within a molecule, converting one isomer into another. These enzymes ensure that all molecules are in the correct form for further metabolic reactions.
An example is phosphoglucoisomerase, which converts glucose-6-phosphate into fructose-6-phosphate in glycolysis. Phosphoglucose isomerase converts glucose-6-phosphate to fructose-6-phosphate in glycolysis and gluconeogenesis.
Significance in Medicine:
Correct isomerization is essential for efficient metabolic flux. Any impairment might lead to abnormal concentrations of metabolic intermediates that affect energy production. In certain cancers and metabolic diseases, anomalous isomerase activity has been observed, making them potential biomarkers and targets for drug development.
Enzyme Inhibition, Kinetics, and Their Clinical Applications
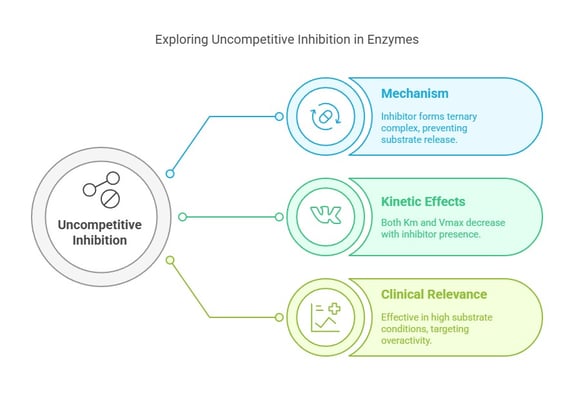
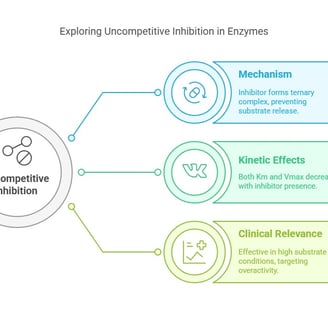
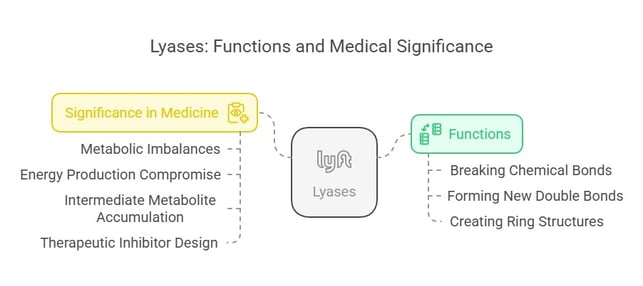
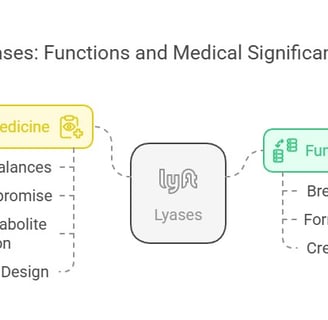
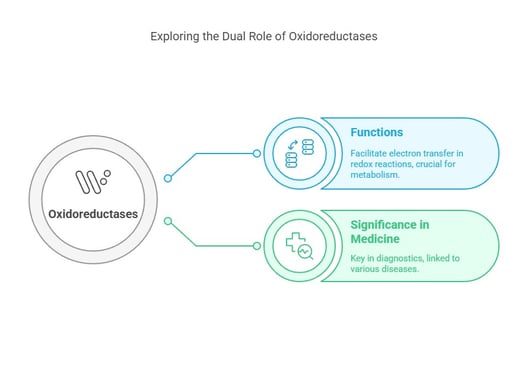
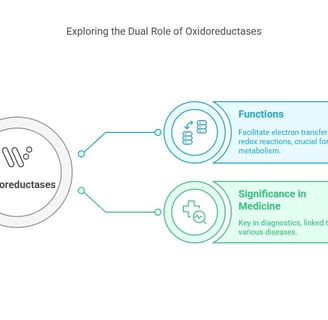
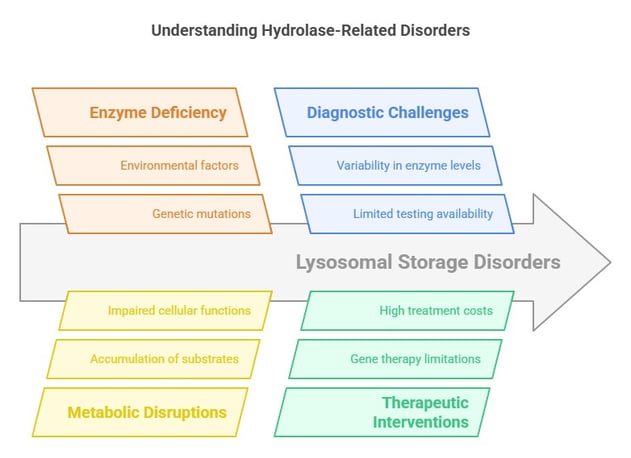
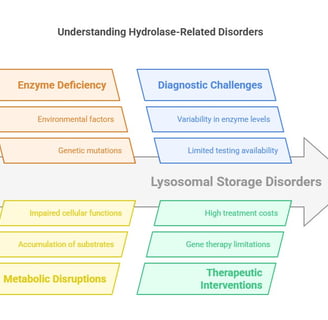
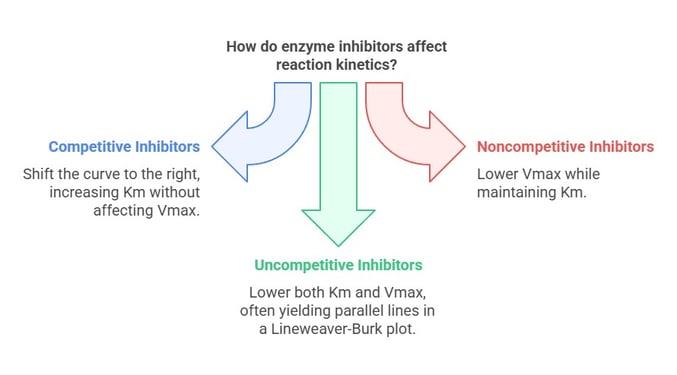
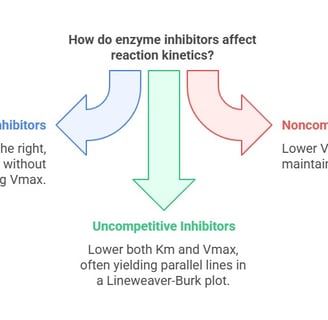
Understanding enzymes is key to grasping how the body’s metabolism is intricately regulated. Enzymes catalyze reactions by lowering activation energies, and their activities can be fine-tuned by various inhibitors and modulated under different physiological conditions. Let us explore the mechanisms behind (1) enzyme inhibition, the foundational (2) principles of enzyme kinetics—particularly (3) Michaelis-Menten kinetics—and how (4 enzyme assays are applied in a clinical context.
Noncompetitive Inhibition
Mechanism:
Noncompetitive inhibitors bind to an allosteric site, which is distinct from the enzyme's active site. This binding can occur whether or not the substrate is bound, often inducing a conformational change that reduces enzyme activity.Kinetic Effects:
Km: It remains largely unchanged since the binding at the active site is not directly competed with by the inhibitor.
Vmax: This decreases because the maximum achievable reaction rate is reduced regardless of substrate concentration.
Clinical & Pharmacological Relevance:
Noncompetitive inhibition is critical in the development of drugs that need to consistently reduce enzyme activity, regardless of substrate fluctuations. This type of inhibition is exploited in some chemotherapeutic strategies where permanent enzyme modulation is desirable.
1. The Mechanisms of Enzyme Inhibition
Enzyme inhibitors are molecules that reduce or halt enzyme activity. They help us understand enzyme structure and function and are critical in drug development by targeting specific metabolic pathways.
Competitive Inhibition
Mechanism:
Competitive inhibitors resemble the enzyme’s natural substrate, binding reversibly to the active site. This prevents the substrate from binding but does not affect the enzyme once the substrate is bound if the substrate concentration is sufficiently high.Kinetic Effects:
Km (Michaelis constant): Increases because a higher substrate concentration is needed to reach half the maximum velocity (Vmax).
Vmax: Remains the same since the inhibition can be overcome with sufficient substrate.
Clinical & Pharmacological Relevance:
Drugs like statins competitively inhibit HMG-CoA reductase, lowering cholesterol synthesis. Competitive inhibitors allow for the modulation of enzyme activity by adjusting substrate levels, making them highly relevant in developing dosage strategies.
Uncompetitive Inhibition
Mechanism:
Uncompetitive inhibitors bind exclusively to the enzyme-substrate complex, not the free enzyme. By forming a ternary complex with the substrate-bound enzyme, the inhibitor effectively locks the substrate in place.Kinetic Effects:
Km and Vmax: Both decrease. The binding of the inhibitor stabilizes the enzyme-substrate complex, effectively lowering the apparent affinity for additional substrates and limiting the maximum reaction velocity.
Clinical & Pharmacological Relevance:
Uncompetitive inhibitors can be particularly effective when the enzyme is in high substrate concentration—conditions often seen in pathologic states. Their unique kinetic profile offers a window in diseases where enzyme overactivity plays a role, allowing for more precise therapeutic interventions.
2. Enzyme Kinetics: Michaelis-Menten Model
Enzyme kinetics helps us understand how enzymes work under varying physiological conditions and how they respond to inhibitors. The Michaelis-Menten model is a cornerstone in this field.
The Michaelis-Menten Equation
The relationship between substrate concentration ([S]) and reaction rate (v) is described by:
[ v = \frac{V_{\max} [S]}{K_m + [S]} ]
(V_{\max}): The maximum reaction velocity is achieved when the enzyme is fully saturated with substrate.
(K_m): The Michaelis constant, which reflects the substrate concentration at which the reaction rate is half of (V_{\max}). It inversely indicates the enzyme’s affinity for its substrate—a lower K_m signifies a higher affinity.
Graphical Representation and Interpretation
Hyperbolic Curve:
When plotting reaction velocity against substrate concentration, the curve approaches (V_{\max}) asymptotically, which is useful for designing experiments and interpreting enzyme behavior.Influence of Inhibitors:
Competitive Inhibitors Shift the curve to the right (increase (K_m)) without affecting (V_{\max}).
Noncompetitive Inhibitors: Lower (V_{\max}) while maintaining (K_m).
Uncompetitive Inhibitors Lower both (K_m) and (V_{\max}), often yielding parallel lines in a Lineweaver-Burk plot.
Importance in Research and Drug Development
Understanding these kinetics not only clarifies enzyme functions but also underpins the development of inhibitors for therapeutic use. Enzyme kinetics provides a quantitative framework for assessing drug efficacy and determining appropriate dosages.
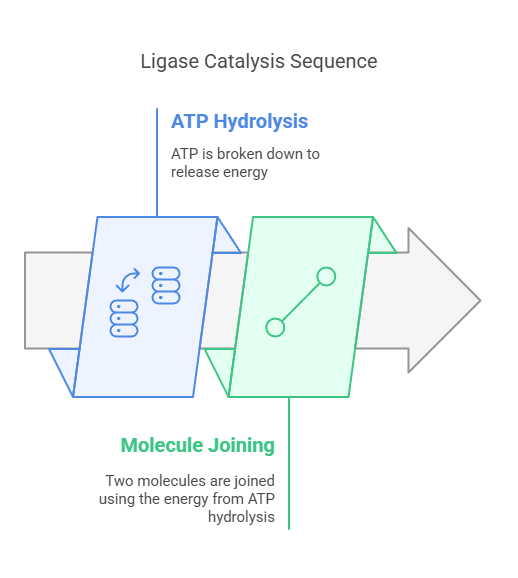
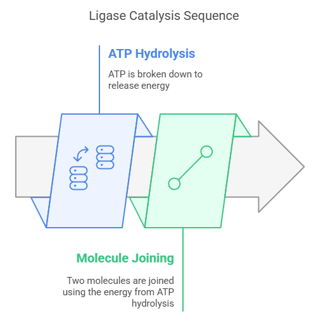
3. Clinical Applications of Enzyme Assays
Enzyme assays are fundamental diagnostic tools in the clinical laboratory. They offer quantitative insights into enzyme activities that help diagnose metabolic disorders, organ dysfunction, and other pathological conditions.
Common Clinical Assays
Liver Enzymes:
AST (Aspartate Aminotransferase) and ALT (Alanine Aminotransferase): Elevated levels indicate liver injury or inflammation.
Cardiac Markers:
CK-MB (Creatine Kinase-MB) and Troponin: These enzymes serve as markers for myocardial infarction.
Pancreatic Enzymes:
Amylase and Lipase: Assays for these enzymes are crucial in diagnosing conditions like pancreatitis.
Metabolic Enzymes:
Glucose-6-Phosphatase, Lactate Dehydrogenase (LDH): Abnormal levels can signal metabolic disturbances or tissue hypoxia.
Making the Connection:
Understanding enzyme kinetics graphs isn’t just an academic exercise; it directly influences how clinicians interpret enzyme assays in real-life situations. By learning to read these graphs and understanding the underlying principles, medical professionals can:
Diagnose Conditions Promptly: Quickly identify pathological changes that indicate conditions like myocardial infarction or liver damage.
Tailor Treatments Accurately: Monitor enzyme activity to adjust drug dosages or intervention strategies for optimum patient outcomes.
Advanced Personalized Medicine: Uses kinetic parameters to understand individual metabolic variations, leading to more personalized care.
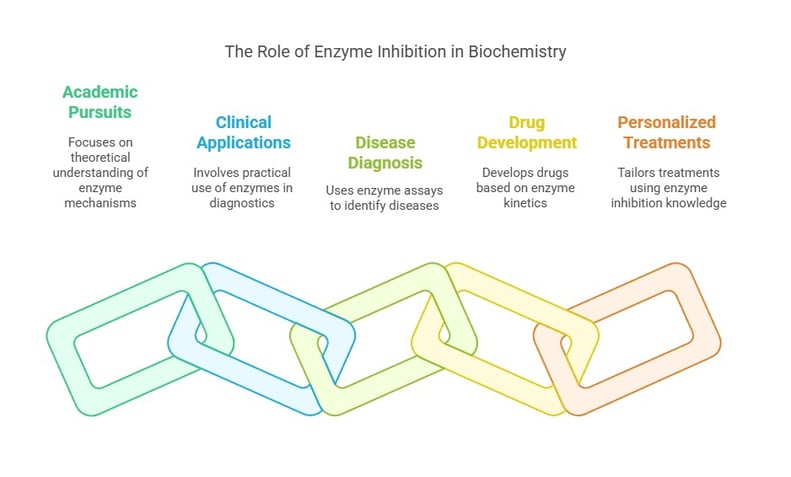
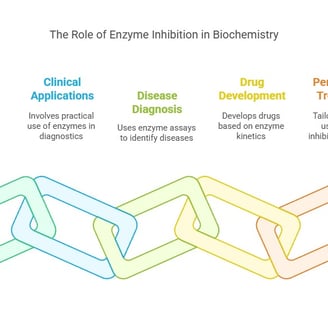
Factors Affecting Enzyme Activity
Several factors influence the activity of enzymes:
Temperature: Each enzyme has an optimal temperature at which it functions most efficiently. Deviations can reduce activity or denature the enzyme, rendering it inactive.
pH Levels: Enzymes have optimal pH ranges. Extreme pH values can alter the enzyme's structure and diminish its activity.
Substrate Concentration: Increasing substrate concentration typically boosts reaction rates up to a saturation point, beyond which there is no further increase.
Enzyme Concentration: Higher enzyme concentrations can increase reaction rates, provided substrate is available.
Inhibitors: Molecules that decrease enzyme activity. They can be:
Competitive inhibitors bind to the active site, blocking substrate access.
Non-competitive inhibitors bind to a different part of the enzyme, altering its shape and function.
Activators: Molecules that increase enzyme activity by enhancing substrate binding or catalytic efficiency.
Factors Affecting Enzyme Activity & Functions
Clinical Aspects of Enzymes
Enzymes have significant clinical applications:
Diagnostic Markers: Levels of specific enzymes in blood or tissues can indicate organ function or damage. For instance:
Alanine aminotransferase (ALT) and Aspartate aminotransferase (AST): Elevated levels may signal liver damage.
Creatine kinase (CK): Increased levels can indicate muscle damage or myocardial infarction.
Therapeutic Agents: Certain enzymes are used as medications. For example:
Streptokinase: Employed to dissolve blood clots in patients with thrombotic disorders.
Functions of Enzymes
Enzymes are indispensable in numerous biological processes, including:
Digestion: Breaking down complex food molecules into absorbable units.
Metabolism: Facilitating pathways that convert nutrients into energy and building blocks for the body.
DNA Replication and Repair: Ensuring genetic information is accurately copied and maintained.
Detoxification: Neutralizing harmful substances within the body.
Their role is critical in maintaining homeostasis and supporting life-sustaining reactions.
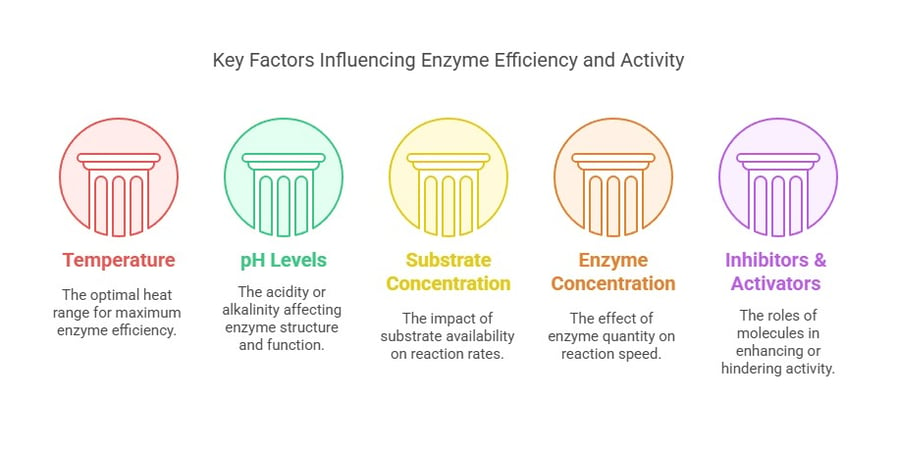
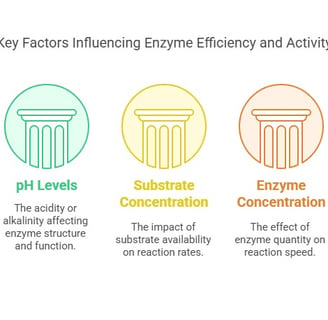
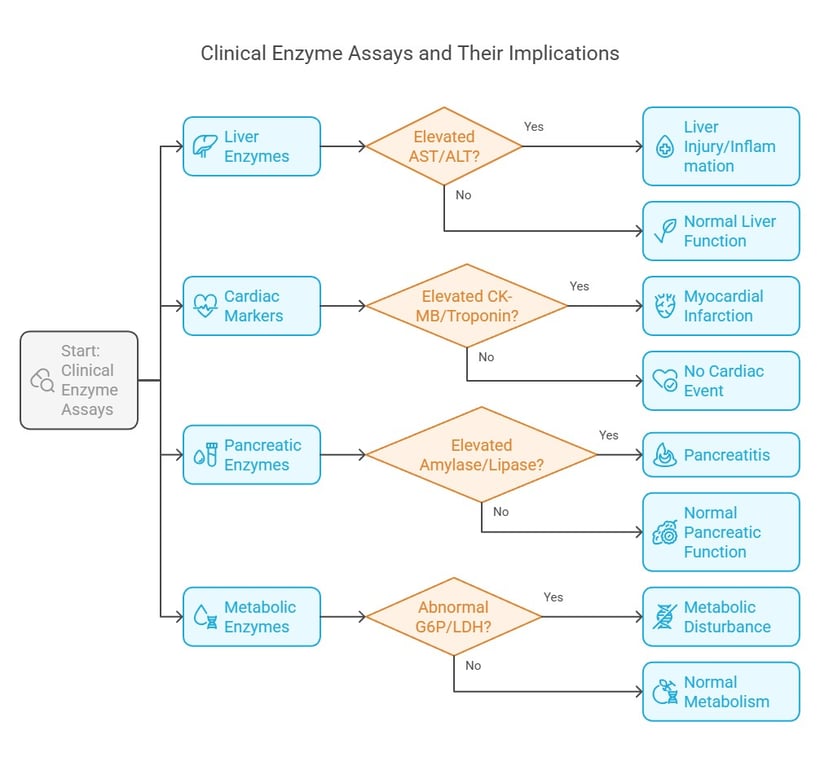
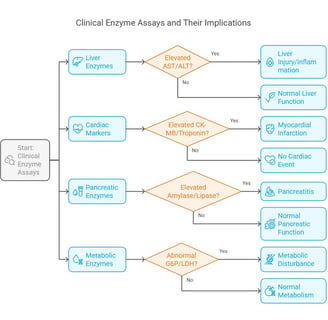
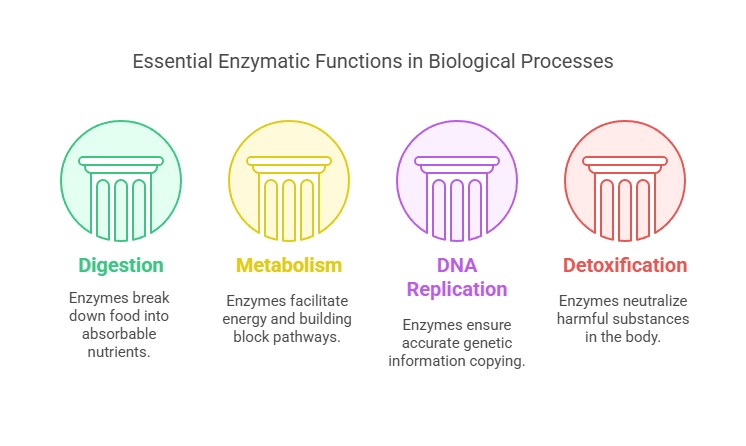
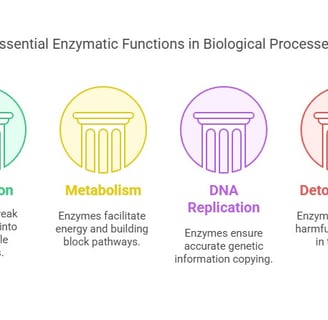
Concluding Thoughts
Understanding the classes of enzymes and their roles in biochemical pathways provides a framework for medical students to appreciate the intricacies of metabolism, physiology, and pathophysiology. Knowledge of these enzyme classes not only aids in grasping normal cellular function but also forms the basis for many diagnostic tests and therapeutic strategies. For example, enzyme inhibitors and activators are routinely employed as drugs to modify metabolic pathways in diseases such as diabetes, cancer, and cardiovascular disorders.
Join us to explore medical biochemistry intricacies.
WRITE TO US
© 2024. All rights reserved.
JOIN OUR NEWSLETTER